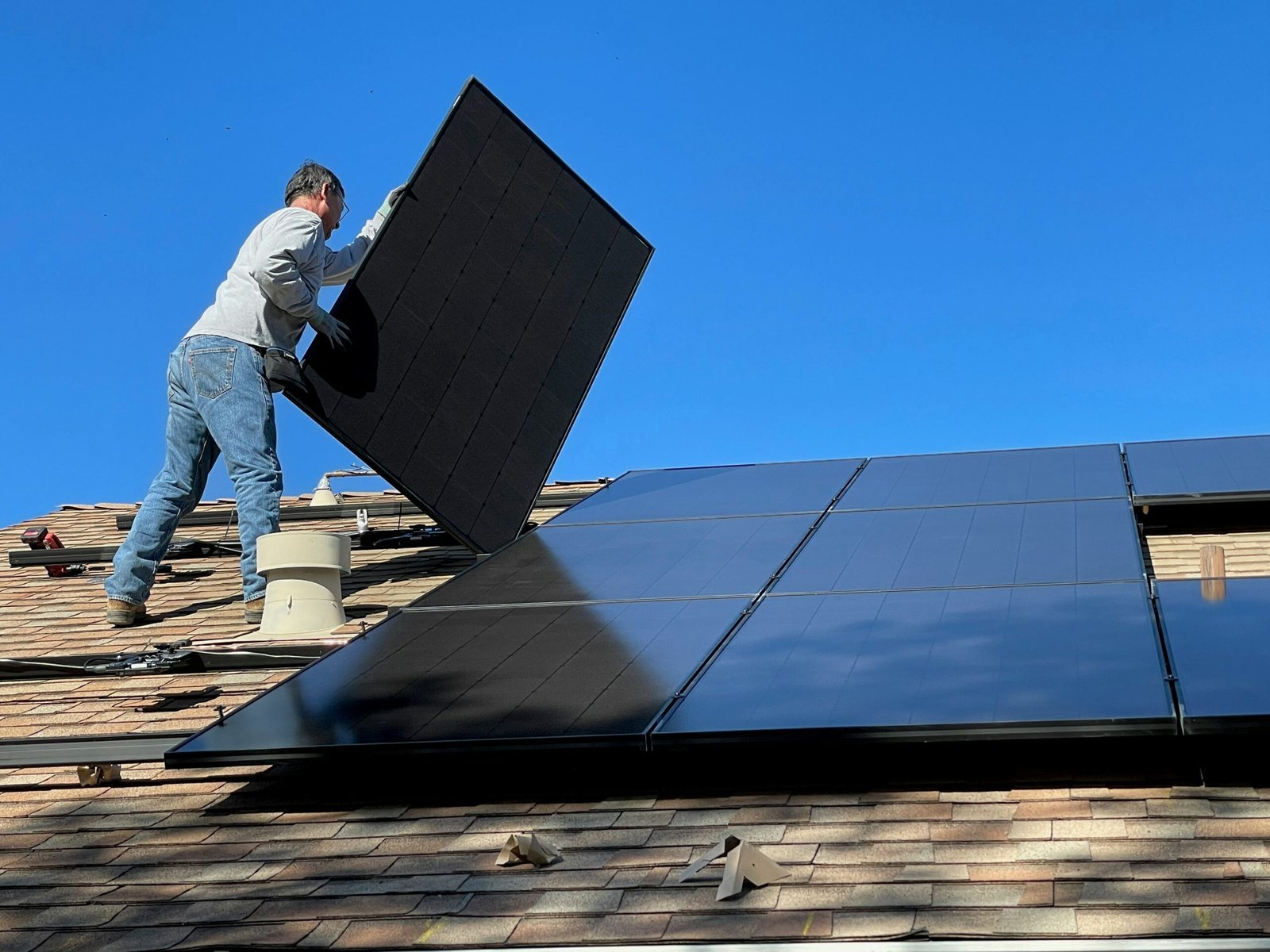
Introduction to the Sun’s Structure
The Sun, a massive ball of plasma, is the central star of our solar system and the most critical source of energy for life on Earth. Its gravitational force keeps the planets, including Earth, in their respective orbits, thereby maintaining the structure of our solar system. The Sun’s radiant energy drives weather, climate, and the cycles of life, emphasizing its indispensable role in our daily existence.
Understanding the Sun involves delving into its complex structure composed of multiple layers, each exhibiting unique characteristics and behaviors. These layers include the core, where nuclear fusion takes place, the radiative and convective zones, and the outer atmospheric layers, namely the photosphere, chromosphere, and corona. Each of these layers contributes distinctly to the Sun’s overall function and the various solar phenomena observed.
Studying the Sun’s structure is crucial for several reasons. Firstly, it helps scientists comprehend the processes that fuel the Sun’s energy production. Secondly, it provides insights into solar activities such as sunspots, solar flares, and coronal mass ejections, which can significantly impact space weather and, consequently, Earth’s technological systems. Furthermore, understanding these layers aids in predicting solar cycles and their effects on Earth’s climate.
In summary, the Sun is not merely a glowing orb in the sky but a complex entity with a layered structure, each layer playing a vital role in its overall function. By studying these layers, scientists can unlock the mysteries of solar phenomena and mitigate their potential impacts on our planet. This detailed exploration of the Sun’s structure lays the foundation for our understanding of the broader cosmos and its influence on life on Earth.
The Core: Heart of the Sun
The core of the Sun, situated at its very center, is the powerhouse driving the immense energy output that sustains our solar system. The core is where nuclear fusion, the process that fuels the Sun, occurs. In this high-energy environment, hydrogen atoms undergo a series of reactions to form helium. This fusion process releases an extraordinary amount of energy in the form of light and heat. As hydrogen nuclei combine, they release energy in accordance with Einstein’s equation, E=mc², which explains how a small amount of mass can be converted into a tremendous amount of energy.
The conditions within the core are extreme by any measure. The temperature reaches approximately 15 million degrees Celsius (27 million degrees Fahrenheit), which is sufficient to overcome the electrostatic repulsion between hydrogen nuclei. At this temperature, the atoms are in a plasma state, where electrons are stripped from their nuclei. The pressure in the core is equally staggering, estimated to be about 250 billion atmospheres, a testament to the immense gravitational forces at play.
Moreover, the density of the core is around 150 times that of water, making it an incredibly compact and energetic region. This density is crucial for maintaining the high pressure and temperature necessary for nuclear fusion to occur continuously. The energy produced in the core takes a convoluted path to the Sun’s surface, eventually radiating into space as the sunlight that warms our planet.
In essence, the core of the Sun is an intricate and dynamic region where the fundamental processes of stellar physics unfold. It is the heart of the Sun, a central engine that not only powers the Sun itself but also provides the energy necessary for life on Earth. Understanding the core’s mechanisms offers profound insights into the workings of our closest star and the broader universe.
The Radiative Zone: Energy Transfer
The radiative zone is a crucial layer of the Sun that lies just outside its core. This region plays a significant role in the transfer of energy produced in the core. In the radiative zone, energy is conveyed outward primarily through the process of radiation. Unlike the convective zone above it, where energy is transported via convection currents, the radiative zone relies on the absorption and re-emission of photons by particles within the dense solar material.
The temperature gradient in the radiative zone is substantial, starting from approximately 7 million degrees Celsius near the core and decreasing to around 2 million degrees Celsius as it approaches the outer layers. This gradient is essential for the continual outward movement of energy. Photons generated in the core embark on a prolonged journey through the radiative zone, constantly being absorbed and re-emitted by electrons and protons in the dense plasma. This zigzagging path significantly delays the photons’ progress, making the energy transfer in this zone incredibly slow.
Due to the high density of the radiative zone, it can take an extraordinarily long time for energy to traverse this layer. Estimates suggest that it may take anywhere from 100,000 to 1 million years for a photon to pass through the radiative zone. This protracted journey is a result of the countless interactions between photons and the solar material, which impede direct travel and necessitate a random walk towards the surface.
Understanding the dynamics of the radiative zone is essential for comprehending the overall energy transfer mechanisms within the Sun. The intricate processes within this zone ensure that energy produced in the core is gradually moved outward, eventually reaching the convective zone where it will be transported to the Sun’s surface and emitted as sunlight. This energy transfer process underscores the complexity and efficiency of the Sun’s internal mechanisms, which sustain the radiant energy that supports life on Earth.
The Convective Zone: Circulating Energy
The convective zone, located directly above the radiative zone, plays a critical role in the Sun’s energy distribution. This layer extends from around 200,000 kilometers below the Sun’s surface up to the visible photosphere. Unlike the radiative zone, where energy transfer occurs primarily through radiation, the convective zone operates on the principles of convection. In this process, hot plasma rises toward the surface, cools as it reaches higher altitudes, and then sinks back down to be reheated. This cycle of rising and falling plasma creates a dynamic environment within the convective zone.
The turbulent motion within this layer is not just a simple up-and-down motion; it involves complex, swirling patterns that contribute significantly to the Sun’s overall behavior. These convective movements are responsible for the granulated appearance of the Sun’s surface, observed as granules or small cells. Each granule can be as large as several hundred kilometers across, highlighting the enormous scale of these convective currents.
This convection process also plays a pivotal role in generating the Sun’s magnetic field. As the plasma moves, it carries along magnetic fields, twisting and tangling them. This activity is part of what drives the solar dynamo, a mechanism that generates the Sun’s magnetic field and leads to various magnetic phenomena. One observable effect of this magnetic activity is the formation of sunspots. These are relatively cooler regions on the Sun’s surface, appearing darker due to the intense magnetic activity that inhibits convection, reducing energy transport to these areas.
The convective zone’s turbulent nature is essential for understanding many of the Sun’s surface phenomena. It is a region where energy from the deeper layers of the Sun is brought to the surface, contributing to the Sun’s complex behavior and observable characteristics. By studying this zone, scientists gain insights into the intricate processes that govern our closest star, enhancing our overall understanding of stellar dynamics.
The Photosphere: The Sun’s Visible Surface
The photosphere is the Sun’s outer shell, the layer that emits the light we observe from Earth. It is essentially the Sun’s visible surface, presenting a fascinating array of features when viewed through a telescope. One of the most notable characteristics of the photosphere is its appearance, which is dotted with granules and sunspots.
Granules are small, bright cells on the photosphere, each about 1,000 kilometers in diameter. These granules are the result of convection currents of plasma within the Sun. Hot plasma rises to the surface, cools as it releases energy in the form of light, and then sinks back into the Sun to be reheated. This dynamic process gives the photosphere a grainy texture, akin to a boiling pot of water.
Sunspots, on the other hand, are temporary phenomena appearing as dark spots on the photosphere. They are cooler regions, with temperatures around 3,800 degrees Kelvin compared to the surrounding areas which are approximately 5,800 degrees Kelvin. Magnetic activity inhibits convection, causing these regions to cool and darken relative to their surroundings. Sunspots are often associated with solar flares and can influence space weather, impacting satellite operations and communications on Earth.
The photosphere plays a crucial role in emitting visible light, which is essential for life on Earth. This layer is also where limb darkening occurs, a phenomenon where the edge of the Sun appears darker than its center. This effect is due to the photosphere’s temperature gradient; we see deeper, hotter, and hence brighter layers at the center, while the edges show shallower, cooler, and dimmer layers.
The photosphere is relatively thin, with a thickness of about 500 kilometers, yet it serves as the critical boundary between the Sun’s interior and its atmosphere. It is through this thin layer that the vast energy generated in the Sun’s core escapes into space, making the photosphere indispensable for understanding solar dynamics and their broader implications.
The Chromosphere: The Sun’s Lower Atmosphere
The chromosphere, situated immediately above the photosphere, represents a significant layer of the Sun’s lower atmosphere. This layer derives its name from the Greek words “chroma” meaning color, and “sphere,” indicative of its spherical shape. During a total solar eclipse, the chromosphere becomes visible as a thin, reddish ring encircling the Sun, a hue attributed to the strong emission of hydrogen alpha light.
One of the most intriguing aspects of the chromosphere is its dynamic and complex nature, hosting various solar phenomena. Among these are spicules, which are narrow jets of rising gas that extend from the lower chromosphere into the upper regions. Spicules, resembling grass-like structures, can reach heights of up to 10,000 kilometers and have lifespans of only a few minutes. Another remarkable feature is the presence of prominences, which are large, bright, gaseous features that often loop hundreds of thousands of kilometers above the Sun’s surface. These prominences are composed of cooler plasma suspended in the hotter outer layers, held in place by magnetic forces.
One of the most notable characteristics of the chromosphere is the significant increase in temperature compared to the photosphere. While the photosphere has a temperature of about 5,500 degrees Celsius, the chromosphere’s temperature climbs dramatically, ranging from 6,000 degrees Celsius at its base to approximately 20,000 degrees Celsius at its outer edge. This temperature gradient plays a crucial role in the dynamics of solar activity, contributing to the formation of the Sun’s magnetic field and influencing solar flares and coronal mass ejections.
The chromosphere is thus a vital component of the Sun’s structure, acting as a bridge between the relatively cool photosphere and the extremely hot corona. Its dynamic interactions and the phenomena it hosts are essential to understanding solar activity and its impacts on the solar system, including space weather effects on Earth.
The Corona: The Sun’s Outer Atmosphere
The corona, the outermost layer of the Sun’s atmosphere, presents a fascinating and somewhat perplexing feature of our closest star. Unlike the layers beneath it, the corona reaches extraordinarily high temperatures, often soaring to millions of degrees Celsius. This phenomenon, known as the coronal heating problem, defies the intuitive expectation that temperatures should decrease with distance from the Sun’s core. Despite extensive research, the exact mechanisms driving this intense heat remain a topic of scientific inquiry, with theories ranging from magnetic reconnection to wave heating.
During solar eclipses, the corona becomes visibly prominent as a striking halo of light encircling the darkened Sun. This ethereal display is possible because the Moon precisely covers the Sun’s bright photosphere, allowing the fainter corona to shine. Its appearance during these rare events has captivated both scientists and the general public, providing invaluable opportunities for studying the Sun’s outer atmosphere.
The corona plays a crucial role in the generation of the solar wind, a stream of charged particles continuously flowing from the Sun into space. This solar wind interacts with planetary magnetospheres and can influence space weather, affecting satellite operations, communication systems, and even power grids on Earth. Furthermore, the study of coronal mass ejections (CMEs)—massive bursts of solar wind and magnetic fields rising above the corona—has become increasingly important. CMEs can have profound impacts on space weather, leading to geomagnetic storms that can disrupt technology and pose risks to astronauts.
Understanding the intricacies of the corona is essential for comprehending the Sun’s behavior and its broader influence on the solar system. Through advanced observational techniques and theoretical models, researchers continue to uncover the secrets of this enigmatic outer layer, shedding light on one of the most intriguing aspects of solar physics.
Conclusion: The Sun’s Layers and Their Significance
Understanding the Sun’s layers is essential for comprehending the complex dynamics that govern our closest star. The Sun is composed of several distinct layers, each with unique characteristics and functions that contribute to its overall behavior and influence on the solar system.
The core is the powerhouse of the Sun, where nuclear fusion generates the immense energy that radiates from the Sun. Surrounding the core is the radiative zone, where energy is transferred outward through radiation. As energy moves further outward, it enters the convective zone, where it is transported by convection currents. Above these, the photosphere is the visible surface of the Sun, emitting the light we see. The chromosphere and the outermost layer, the corona, both play crucial roles in solar phenomena such as solar flares and coronal mass ejections.
Comprehending these layers is pivotal for understanding solar dynamics, which have profound effects on space weather and the broader solar system. For instance, solar flares and coronal mass ejections can impact satellite operations, communication systems, and even power grids on Earth. By studying the layers of the Sun, scientists can better predict these solar activities and mitigate their impacts.
Ongoing research and missions continue to unveil more details about the Sun’s intricate structure and behavior. Missions like NASA’s Parker Solar Probe and the European Space Agency’s Solar Orbiter are designed to gather unprecedented data by venturing closer to the Sun than ever before. These missions aim to provide deeper insights into the mechanisms driving the Sun’s activity, enhancing our understanding and enabling more accurate space weather forecasting.
In conclusion, the layers of the Sun are integral to its function and influence on the solar system. Through continued scientific inquiry and advanced space missions, our knowledge of the Sun’s structure and behavior will continue to grow, offering greater protection for our technological infrastructure and enriching our comprehension of this vital celestial body.